When we think of parasites, the image is often insect-like, with lots of legs and beady eyes and mean-looking pincers. However, the most dangerous parasites are actually microscopic, and they include trypanosomes, Plasmodium (which causes malaria), and roundworms (like Ascaris).
According to the Global Burden of Disease Study, over one million deaths could be attributed to parasitic diseases in 2013 alone, with malaria taking the number one spot followed by leishmaniasis. Since then, huge strides have been made in terms of control measures. However, these parasites still pose a huge danger to human health as millions are still at risk around the world.
Introduction to the Leishmania parasite
Leishmania is a genus of intracellular protozoan parasites, meaning that this is a type of parasite that hides inside the cells of the host. It is transmitted between hosts via female sandfly vectors, which are insects classified into the Phlebotominae subfamily. In the Americas (also known as the ‘New World’), the genus of sandfly that spreads Leishmania is Lutzomyai. Whereas in Eurasia (‘Old World’), the main genus is Phlebotomus.
The class that Leishmania belongs to is known as Kinetoplastea. Members of this class are characterised by the presence of a kinetoplast, which is an organelle containing multiple copies of mitochondrial DNA. Kinetoplastids also have at least one flagellum with an invagination at the end. Another example of a kinetoplastid is Trypanosoma brucei, the parasite which causes African trypanosomiasis (commonly known as ‘sleeping sickness’).

Figure 1: The structure of Leishmania parasites. The promastigote form occurs in the sandfly vector and contains a flagellum for locomotion. On the other hand, the amastigote form occurs in the host and is non-motile. Figure credit goes to Designua.
Despite being classified into the same genus, Leishmania parasites are quite diverse. In fact, the Leishmania genus is further divided into four subgenera: Leishmania, Mundinia, Sauroleshmania, and Viannia. The subgenera are characterized by the different development sites in the mosquito vector. These subgenera are then divided into different species.
Of the several species of Leishmania, most species are zoonotic, whereby a zoonotic disease (zoonoses) is one which ‘jumps’ from an animal to a human. These animals, in particular, are known as ‘reservoir hosts’ and play an important role in Leishmania transmission. One of the common reservoir hosts for Leishmania is the domestic dog.

Figure 2: The transmission cycle of Leishmania parasites. An infected sandfly will inject non-dividing Leishmania promastigotes into a non-infected host during a blood meal. Once within the host, the promastigotes will differentiate into amastigotes after invading the host cells. An uninfected sandfly can become infected when taking a blood meal from an infected host. The sandfly will ingest amastigotes from the infected host, which then differentiate into procyclic promastigotes (i.e. cells which are capable of dividing).
Leishmaniasis: symptoms and epidemiology
More than 20 species of Leishmania can infect humans alone, causing a disease known as leishmaniasis. Leishmaniasis is a neglected tropical disease and remains prevalent in over 98 countries, thereby posing a heavy burden on poorer populations specifically.
There are two main types of leishmaniasis: Cutaneous and Visceral.
Cutaneous leishmaniasis causes lesions on the body and face, but the infection remains confined mainly to one area. It is caused primarily by the L. major or L. tropica species in Eurasia and the L. mexicana species in the Americas. Though, despite this disease being mainly prevalent in the Middle East and South America, cases can still pop up in any tropical climate. Meanwhile, a similar condition called mucocutaneous leishmaniasis occurs when a mucosal surface (such as the inside of the nose) is infected.
On the other hand, visceral leishmaniasis is a chronic and systemic (meaning it spreads throughout the entire body) illness that causes mucosal ulcers, anaemia, fever, and the enlargement of our liver and spleen. This dreadful disease is caused by the species L. donovani, and is most prevalent in South Asia (Afghanistan, India, etc.) and Brazil. Besides that, it is also found in parts of North and East Africa.
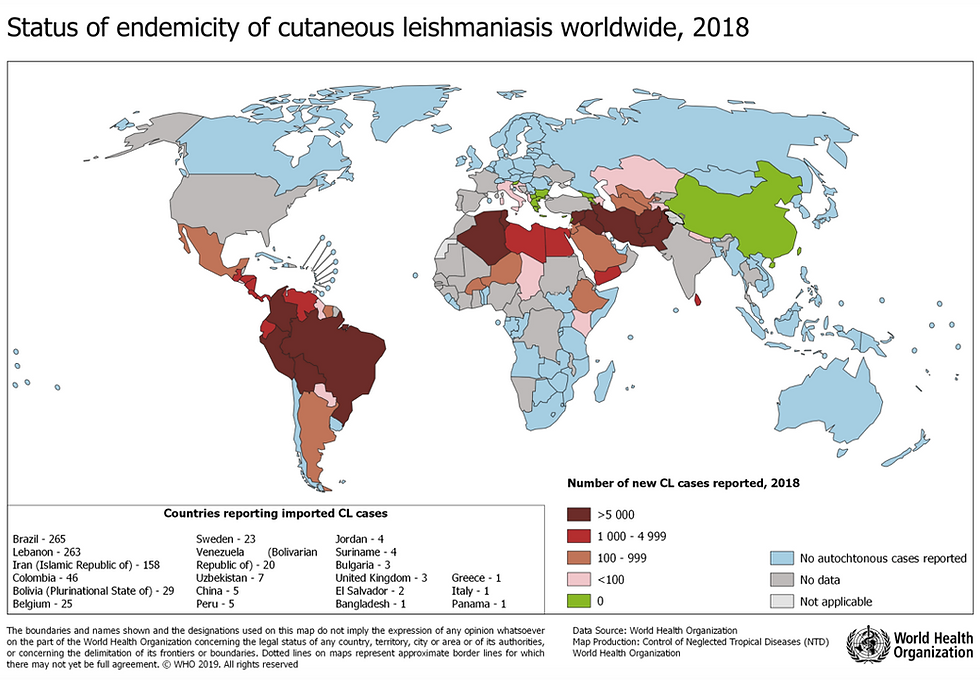
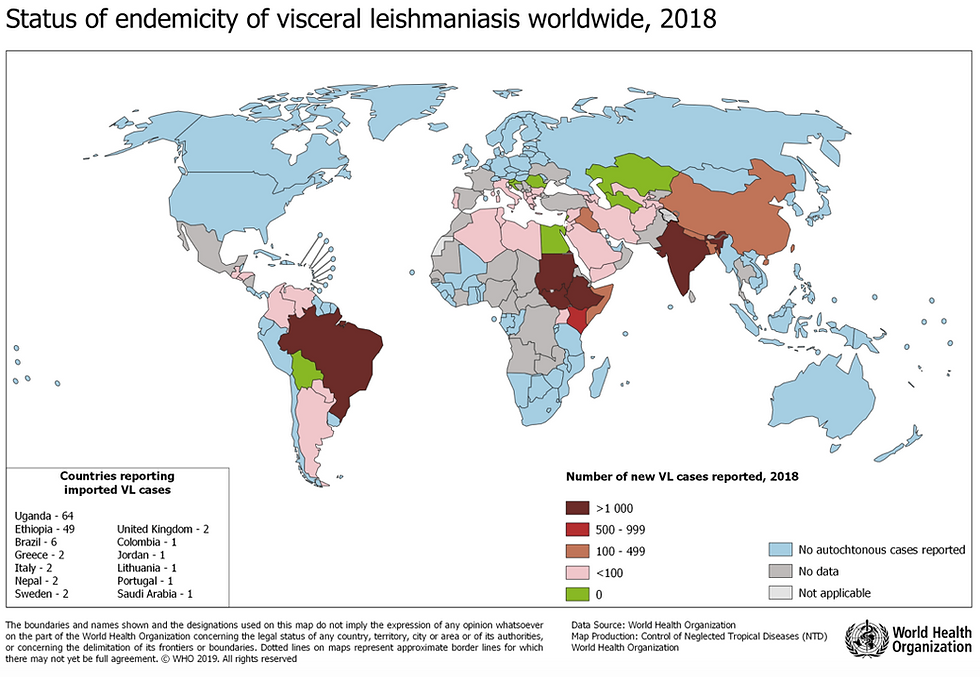
Figure 3: The epidemiology of leishmaniasis (as of 2018). India, North Africa, and Brazil remain prime hotspots for cutaneous and visceral leishmaniasis. Image courtesy of the World Health Organisation.
How does Leishmania attack our body?
When an infected female sandfly takes a blood meal from a mammal host, it releases Leishmania promastigotes into the bloodstream via its saliva. Soon after, the complement system, which is a part of our innate immune system, deposits a protein called C3b on the parasite surface.
The presence of C3b signals to a type of immune cell known as macrophages that the parasite is a foreign entity and must be disposed of. The macrophages then engulf the C3b-coated promastigotes in a process called phagocytosis.
However, this is exactly what Leishmania wants!
Normally, the complement system will destroy an invading pathogen via the formation of the membrane attack complex (MAC). The MAC is a complex of different complement proteins which interfere with the cell membrane of a pathogen, causing lysis and cell death - it’s almost like a cellular drill that pokes a hole into the membrane!
However, Leishmania promastigotes are able to form a shield against the MAC and other complement proteins. The key to this protection is the dense layer of proteins called lipophosphoglycan (LPG) on the promastigote surface. This LPG ‘coat’ protects the parasite from the lytic properties of the complement system, thus allowing it to survive phagocytosis. In other words, the promastigote form of Leishmania actually parasitizes (i.e. infects) the macrophages, and this invasion of macrophages is what causes the clinical symptoms of leishmaniasis.
Besides that, it is also important to know that the macrophage can actually engulf the parasite in several different ways.
The most straightforward way is direct phagocytosis - the promastigote could be directly engulfed by a macrophage. Alternatively, the parasite could first be engulfed by a neutrophil, another innate immune cell. Within the neutrophil, the parasite will induce apoptosis (i.e. programmed cell death). The apoptotic neutrophil will release a signal, and the macrophage will engulf the dying cell. In this manner, the neutrophil acts like a ‘Trojan horse’, delivering the Leishmania promastigote to the macrophage. Thus, Leishmania will get to where it wants to be.
Normally, the macrophage will engulf a cell into a phagosome, and this phagosome will fuse with the macrophage’s lysosome to form the phagolysosome, which is a cell compartment filled with many lytic enzymes that can cause the destruction of the cell components. However, Leishmania promastigotes are capable of remodelling the phagosome to delay the formation of this phagolysosome!
Once engulfed, the parasite begins remodelling the phagosome into a parasitophorous vacuole (i.e. a compartment where the parasite can safely survive within the host cell). A critical characteristic of this remodelling is the LPG coat. As a result, the parasite remains protected within the host cell.
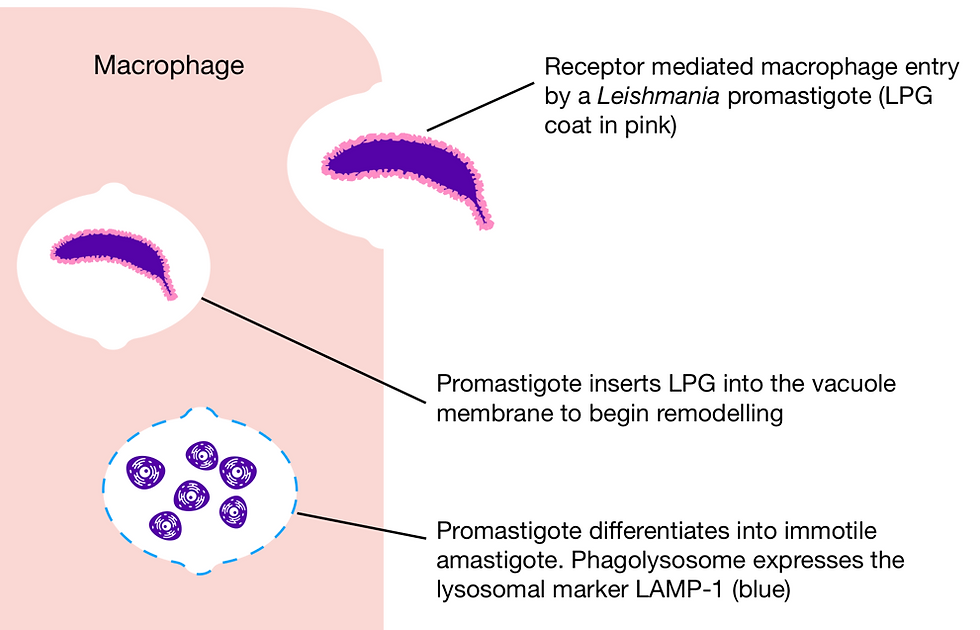
Figure 4: Macrophage invasion by Leishmania parasites. The LPG coat that the parasite clad itself with prevents the formation of the membrane attack complex. Complement proteins attached to the promastigote are detected by receptor proteins (e.g. the CR1 receptor detects C3b) on the surface of the macrophage. The promastigote remodels the parasitophorous vacuole by inserting LPG molecules into it. Finally, the amastigote form develops within the vacuole, and the vacuole is fully remodelled to express host lysosomal markers such as LAMP-1. This fools the host cell into thinking that the parasitophorous vacuole is actually a regular lysosome.
Unfortunately for the parasite, phagolysosome formation cannot be completely stopped by promastigotes - only delayed. As the temperature and acidity levels rise (i.e. pH reduces), the Leishmania promastigote differentiates into the amastigote form. Amastigotes feel perfectly at home in the hostile environment of the phagolysosome. In fact, their metabolism is optimized for acidic pH (around pH 4.0). So, the amastigotes would replicate asexually within the parasitophorous vacuole until the cell bursts, and then stream out to infect other macrophages.
In the meantime, you may be wondering - how does this process differ between cutaneous and visceral leishmaniasis?
In cutaneous leishmaniasis, the infection is confined to mainly one area. As amastigotes replicate and burst out, the accumulated cellular debris starts clogging the blood vessels and thereby cutting off the blood supply. As a result, this causes the infection site to ulcerate (i.e. develop into an ulcer).
On the other hand, in visceral leishmaniasis, the amastigotes infect cells of the mononuclear phagocyte system (MPS), which consist mainly of blood monocytes and tissue macrophages. These cells circulate through organs of the MPS (liver, spleen, lungs) via the lymphatic system, where the Leishmania infection can then spread to. This is far more dangerous as the tissue of the liver and spleen can become infected.
How does the body defend itself against Leishmania?
Now that we’ve taken a look at Leishmania’s tactics in infecting host cells and establishing an infection, let’s see how our immune system fights back!
As mentioned earlier, the innate immune system is the first to encounter Leishmania. In particular, the complement system deposits proteins on the parasite surface, but Leishmania prevents complement-mediated lysis by the presence of its LPG coat. Despite this, all hope is not lost, as the alternative complement pathway remains capable of lysing parasite cells.
Neutrophils also engulf Leishmania, but the parasite hijacks these cells as a way to enter macrophages. Nevertheless, we still have the natural killer (NK) cells on our side, and they are a critical component of the innate immune system that has the ability to lyse parasite cells. In essence, NK cells are cytotoxic and they release granzymes and perforins into the parasite cell. These enzymes have lytic effects and can also disrupt the cell membrane, eventually causing cell death. More importantly, NK cells also produce interferon-γ (IFN-γ), a type of cytokine that plays a critical role in the clearance of parasites (more on this later!).
Meanwhile, dendritic cells - another component of the innate immune system - are a type of antigen-presenting cell (APC) that pick up Leishmania antigens and expose them on their surface. These cells secrete interleukin-12 (IL-12) which signals CD4+ T cells (part of the adaptive immune system) to differentiate into T-helper type-1 (TH1) cells.
Following that, TH1 cells secrete IFN-γ, which stimulates infected macrophages to produce an enzyme known as nitric oxide synthase (NOS). This enzyme generates nitric oxide, which then kills the parasites and thus helps to clear out the nasty infection!

Figure 5: Immune signalling in response to Leishmania infection. This image was adapted from Ikeogu et al. (2020)’s Leishmania Immunity: Advancing Immunotherapy and Vaccine Development.
How Leishmania continues to evade our immune system
Despite the efforts of our immune system, Leishmania has many more tricks up its sleeve to facilitate its survival. For example, we’ve talked about how the LPG coat is critical for Leishmania’s defence against the complement system as well as the phagolysosome.
Other than that, Leishmania species also alter host cell signalling to interfere with the immune system’s response. Generally speaking, infected macrophages defend themselves by producing reactive oxygen species (ROS) through the NADPH oxidase complex. These ROS are capable of causing cellular damage and a so-called ‘oxidative burst’ to prevent the spread of the parasite to even more cells. Leishmania disrupts the formation of ROS by blocking a signalling pathway known as the protein kinase C signalling, which is essential to activate the NADPH oxidase complex. Besides that, Leishmania also deactivates another signalling pathway known as the JAK-STAT signalling pathway, which is needed for activating the secretion of IL-12 and IFN-γ.
And as if that wasn’t enough, Leishmania can also induce the production of certain chemokines and cytokines which facilitate parasite survival, such as CCL7 and IL-10. Some of these factors induce the differentiation of CD4+ T cells into T-helper type 2 (TH2) cells (refer Figure 5).
Basically, CD4+ T cells can differentiate into either TH1 or TH2 cells, and each of them is associated with a different type of immune response. In the case of Leishmania, a TH1 response results in parasite killing, while a TH2 response is strongly associated with parasite survival. As shown in Figure 5, TH2 cells inhibit the actions of TH1 cells and make them less effective - consequently, the suppression of TH1 cells leads to a reduction in IFN-γ signalling, which means fewer parasites are killed via the NOS mechanism.
As you can probably tell at this point, Leishmania has a myriad of ways to facilitate its survival in the host by both avoiding and suppressing components of the innate and adaptive immune system.
All in all, this makes it both a dangerous pathogen as well as an intriguing topic of research.
Current prevention and treatments for leishmaniasis
Prevention
Leishmaniasis is mainly prevented by suppressing and killing sandfly vectors which transmit the disease. These are known as vector control measures. As there are around 70 species of sandfly known to transmit Leishmania parasites to humans, vector control measures are critical to reducing disease incidence in the absence of an effective vaccine.
The primary strategies of vector control are indoor residual spraying (IRS) and long-lasting insecticide-treated nets (LLINs). IRS involves spraying the walls and other surfaces of houses and buildings with insecticides to repel and discourage vectors from that area. In contrast, LLINs offer protection to individuals when they are sleeping at night, as that is when sandflies are most active.
Originally, DDT (dichloro-diphenyl-trichloroethane) was the main insecticide used for vector control, but it has since been replaced with pyrethroid insecticides. The reason for that is because pyrethroid insecticides have been proven to be useful in controlling both sandflies and other vectors such as the Anopheles mosquitoes, which transmit malaria. In essence, IRS and LLINs utilise pyrethroids and are effective methods as they are easy to implement, relatively cheap, and quite effective when used correctly.
Treatments
Cutaneous leishmaniasis can often rectify itself without treatment. However, mucocutaneous and visceral leishmaniasis are more dangerous, and if the patient is left unattended, the outcome can often be fatal.
The main treatments for leishmaniasis are pentavalent antimonials, which are drugs containing the element antimony (Sb). The two primary pentavalent antimonials used are sodium stibogluconate and meglumine antimoniate.
Despite being the first line of treatment, antimonials have many disadvantages – they are expensive and can only be administered by intravenous injection. Besides that, they also cause a huge range of side effects and may even be toxic to the host. More importantly, drug resistance has rendered antimonials largely ineffective without the use of additional drugs and combination therapies.
There are other options for antimonials, such as Amphotericin B and miltefosine. Though unfortunately, these drugs can also exhibit toxicity. Furthermore, despite these drugs often being used in combination with antimonials, drug resistance has been seen against combination regimens.
In light of the above, a more promising 2018 review revealed that combining pentavalent antimonials with alternative therapies resulted in a generally high cure rate (70 to 100%) for cutaneous and mucocutaneous leishmaniasis. Despite these hopeful results, more stringent clinical trial data is needed to evaluate the true effectiveness of these combinations.
Efforts towards a leishmaniasis vaccine
Currently, no widely effective vaccine exists for human leishmaniasis, although two vaccine candidates are currently in clinical trials. And on a more positive note, there is one licensed vaccine for canine leishmaniasis called Leishmune currently used in Brazil.
Moving forward, there are many different types of vaccines that we could potentially develop for leishmaniasis:
These vaccines consist of live but attenuated (i.e. weakened) parasites so that they do not cause a full-blown infection. Usually, researchers would use chemicals or irradiation to weaken these parasites. Whilst live attenuated vaccines are known to induce a good immune response to the parasites, the risk of vaccine-induced infection, however, is still very much present. That being said, a live attenuated vaccine is currently available and in use in Uzbekistan.
As the name suggests, killed vaccines contain dead parasites, which means there is no risk of vaccine-induced infection. On the plus side, these vaccines are less expensive to produce, but they do not induce the same level of immune response as a live attenuated vaccine.
A fractionated vaccine basically uses a specific fraction of the parasite containing the antigens required to generate an immune response.
A great example of this would be the Brazilian vaccine for canine leishmaniasis, Leishmune®. This is a fractionated vaccine containing a protein subunit from Leishmania donovani. Interestingly, Leishmune® was also demonstrated to have the potential to block transmission of Leishmania parasites between infected and uninfected dogs via sandfly bites (in this case, the infected dogs have been given the vaccine, which then prevents the disease from spreading to uninfected dogs). This transmission blocking potential is critical to stop the spread of the disease between reservoir hosts.
One important thing to note - transmission blocking vaccines (TBVs) are unlike traditional vaccines as instead of protecting the already-infected host, they protect uninfected hosts from then being infected. In this manner, TBVs have the potential to stop transmission of various vector-borne diseases such as leishmaniasis, malaria, and dengue fever.
Recombinant vaccines are made by using an expression system to produce antigens. An expression system is a modified organism (such as bacterial or mammalian cells) which is engineered to express a gene of interest. The cell then transcribes and translates the gene into a protein which can then be used in downstream applications. In other words, the cells are engineered to produce the desired Leishmania antigens. Following that, the antigen proteins are then purified from the cells and used in a vaccine.
Leish-111F/MPL-SE is a recombinant vaccine candidate currently in phase II trials. The Leish-111F component consists of three proteins fused together (two proteins from L. major, and one from L. braziliensis), while the MPL-SE component is an adjuvant – a molecule specifically designed to increase the efficacy of the active component of the vaccine.
As the name suggests, an antigen cocktail vaccine utilises a mixture of several antigens to elicit strong protection.
So far, there has been some progress in using antigen cocktails to produce immunity against canine leishmaniasis in vitro (i.e. in a lab, not in a live dog). More specifically, the antigen cocktail produced a Th1 response, which as we mentioned earlier is critical for parasite clearance.
6. DNA vaccine
In essence, DNA-based vaccines contain the DNA encoding specific Leishmania antigens, which can then be transcribed and translated by the host cellular machinery. The resultant protein is detected by innate immune cells - such as antigen presenting cells - and expressed on their surface. Once that has occurred, the immune system can then detect and act upon the exposed antigens in the same way as described in the earlier sections.
A 2006 study showed that a DNA vaccine of ten Leishmania antigens elicited a TH1 response in inoculated foxhounds. The dogs were given the vaccine and then challenged with live L. donovani promastigotes, and the results showed significant parasite clearance. Such in vivo studies are a big step towards a working canine and human leishmaniasis vaccine.
Notably, a 2011 study later showed that DNA vaccines boosted with a protein vaccine showed longer lasting protection in susceptible mice. This, therefore, showed how different vaccine development techniques can be used in tandem (meaning ‘together’) to achieve the desired result.
Challenges in vaccine development
With close to one million new cases a year, the need for a leishmaniasis vaccine is overwhelming. Unfortunately, developing a leishmaniasis vaccine has several unique challenges.
The first challenge is cost. To put it simply, vaccine development is extremely expensive, and the total bill could go up to approximately $300 million per vaccine development pipeline. As a result, many industry leaders are often reluctant to put money and research efforts into new vaccines unless there is scope for profit.
Since leishmaniasis is mainly endemic to developing countries, there is less profit incentive for private companies to devote resources towards a vaccine. As a result, the main source of research funding for neglected tropical diseases such as leishmaniasis comes from charities such as the Bill & Melinda Gates Foundation.
In addition to that, finding a vaccine candidate that is both highly immunogenic (i.e. elicits a strong immune response) and provides long-lasting immunity is - in two words - very difficult. Unfortunately, no single candidate has been able to fulfil both criteria well enough so far. Not to mention, this is made even more difficult by the wide variety of Leishmania species which can often have very different genetic profiles despite causing the same diseases.
Concluding remarks: why should we pay attention to Leishmania?
After delving into the details of leishmaniasis and the challenges associated with its prevention, treatment, and prophylaxis, you should hopefully now have a better idea of how burdensome this disease truly is.
In developing countries where leishmaniasis is prevalent, this burden is even more apparent. Poor sanitation and lack of secure housing infrastructure exposes more people to the parasite vector and increases the likelihood of developing a severe infection. What’s more, the lack of health facilities in remote or poorer areas exacerbates the manifestation of the disease as infected individuals do not receive the appropriate treatment when they should. Additionally, current medications for leishmaniasis are extremely expensive, so they pose a huge financial burden on infected individuals and their families.
Consequently, this creates a cycle of illness and poverty that disrupts an affected nation’s ability to develop economically. Aside from that, even though many great strides have been made in terms of vector control, vector and parasite drug resistance continue to threaten or even reverse the progress that’s being made. This is especially concerning since we do not have an effective vaccine as of yet.
As a concluding remark, one additional way to tackle the situation in endemic areas is to make information about the vector and the disease widespread in a way that lay people can understand easily. Thus, everyone would become much more vigilant and play a more active role in preventing the spread of leishmaniasis.
Author
Devyani Saini
MRes Molecular & Cellular Biosciences
Imperial College London
Comments