Vitamin A deficiency – a global issue
Malnutrition bears an infamous reputation as a global health issue. In particular, Vitamin A deficiency (VAD) is a prevalent problem in developing countries, where the low-income population group takes the worst hits. Much of this is attributed to their diets mainly consisting of rice, which is a less nutritional staple food, as a result of lacking purchasing power and awareness.
The consequence of leaving VAD untreated can result in serious adverse health consequences such as suffering from night blindness, Xerophthalmia, and Bitot spots, as reported by The United Nations Children's Fund (UNICEF). In fact, VAD is the main culprit of preventable childhood blindness amongst young children living in developing nations.
A 2009 report by the World Health Organization (WHO) classified 122 countries with a VAD problem at a moderate to severe level, with the highest proportions of those countries located in the African (48%) and South-east Asian (44%) regions in 2013. 5.3 million children and 9.8 million pregnant women (in 88 countries) risk contracting VAD. In Bangladesh, VAD was the culprit of annual 0.2 to 0.5% loss in Gross Domestic Product (GDP).
What is genetic engineering?
Genetic engineering (occasionally referred to as genetic modification) can be defined as a type of technology that directly manipulates DNA (deoxyribonucleic acid) to amplify or alter a specific phenotypic characteristic of an organism. Some examples of the possible modifications include changing a single base pair (i.e. A=T or C≡G), introducing extra copies of genes, eliminating an entire section of DNA, or even cross-combining the DNA from the genomes of two different organisms.
Genetic engineering has been primarily applied in agriculture to improve the nutritional content, resilience, yield and growth rates of many different types of crops such as rice, potatoes, and tomatoes.
Introducing the Agrobacterium-mediated plant transformation technology
Agrobacterium tumefaciens is a soil bacterium that infects both monocotyledonous and dicotyledonous plants, causing crown gall disease.
A. tumefaciens has the ability to incorporate new genetic material into a target plant cell. To dive deeper into the details, the introduced genetic material is called the transferred DNA (T-DNA). This T-DNA is found within a tumour-inducing (Ti) plasmid, which is a circular piece of DNA present in nearly all types of bacteria. During the process of transformation, numerous components of the Ti plasmid allow the effective introduction of new genes into the target cell. These components include:
1. The left and right border sequences of T-DNA
These borders have an established polarity and permit our gene of interest to be cloned into the Ti plasmid.
2. The virulence (vir) genes
These vir genes encode vir proteins, which play an essential role in helping the T-DNA region to be transferred into the target plant genome.
3. The T-DNA region that can be modified
This region of the plasmid, which originally contains the oncogenes that cause the formation of tumours (crown gall disease), can be modified to include our genes of interest as a replacement.
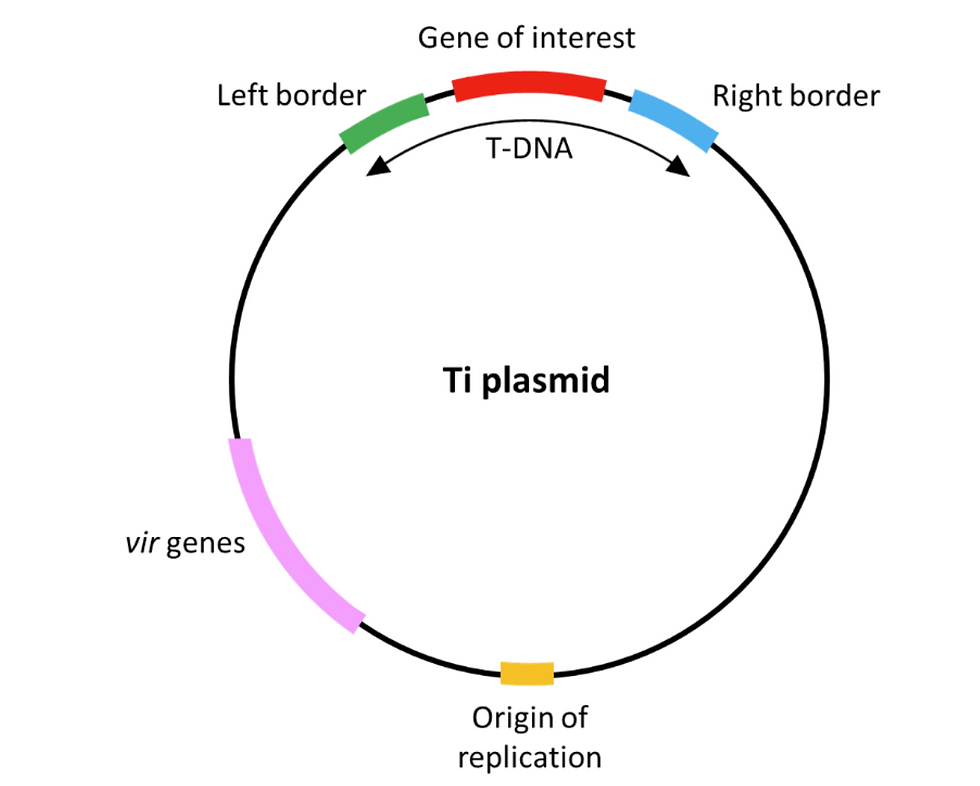
Figure 1: A simplified diagram of the Ti plasmid. This figure illustrates the components of the T-DNA, vir genes as well as the origin of replication (i.e. the specific DNA sequence where DNA replication starts).
With this knowledge in mind, the properties of A. tumefaciens are exploited in the five-step Agrobacterium-mediated transformation process. This process involves wounding the chosen host plants and then soaking them in a solution containing Agrobacterium along with the gene of interest. The injured plant cells release acetosyringone and sugars. These are signals recognised by proteins that in turn signal the assembly of the type IV secretion system (a protein complex found in prokaryotes; used to transport DNA). This allows the transfer of DNA and several vir proteins into the host plant cell. Upon entry into the host cell, vir proteins guide T-DNA into the nucleus, and the genome of the host plant gets genetically modified by the newly inserted DNA.
In the present day, Agrobacterium-mediated transformation is the most popular genetic engineering technique for plants due to its relatively high success rate. Much of this efficiency can be accredited to this technique being able to stably integrate a transgene (which means a newly introduced gene) into a target plant genome without causing any significant damage.
Applying this technology for the Golden Rice Project
Agrobacterium-mediated transformation can be utilised to improve the nutritional content of food, with the Golden Rice being one of the most notable applications of this technique. This was a solution designed to address the problems of Vitamin A deficiency (VAD) predominantly in South-East Asians and African countries, which have the highest rice consumption.
Golden Rice is a biofortified crop, meaning that its nutritional value is enhanced via either conventional plant breeding schemes, agronomic practices, or biotechnological innovations. This idea was first proposed back in 1999, where Prof. Ingo Potrykus and Prof. Peter Beyer successfully secured research funding from a charitable institution known as the Rockefeller Foundation to genetically modify the nutrient content in rice.
The original version of Golden Rice (SGR1) was created to increase the β-carotene (provitamin A) content in the endosperm of rice, by transforming it with the psy gene from daffodils (scientifically named as Narcissus pseudonarcissus) and the crtl gene from a species of soil bacterium called Pantonoea ananatis (formerly Erwinia uredovora). Primarily, the psy and crtl genes encode enzymes that are important for catalysing the formation of β-carotene.
The process kickstarts with inserting these two genes into a Ti plasmid and incorporating it into Agrobacterium. The Agrobacterium is then mixed with rice embryos in a Petri dish, where the rice-embryo containing β-carotene genes are selected and grown into adult plants (these now have β-carotene in their endosperm). These two enzymes undergo a four-step process to catalyse the formation of β-carotene, giving the Golden Rice its distinctive yellow colour.
Upon consuming Golden Rice, β-carotene will be converted into Vitamin A during metabolism, and this Vitamin A will be used by our body to maintain healthier skin, immune system as well as vision.
An improved version of Golden Rice (SGR2) analogous to SGR1 was developed in 2005, where the psy gene isolated from daffodils was replaced with the same gene from maise. Both versions undergo the same transformation procedure to form Golden Rice, but SGR2 has 23 times the total carotenoid content than SGR1 (β-carotene remains as the predominant form of carotenoid).
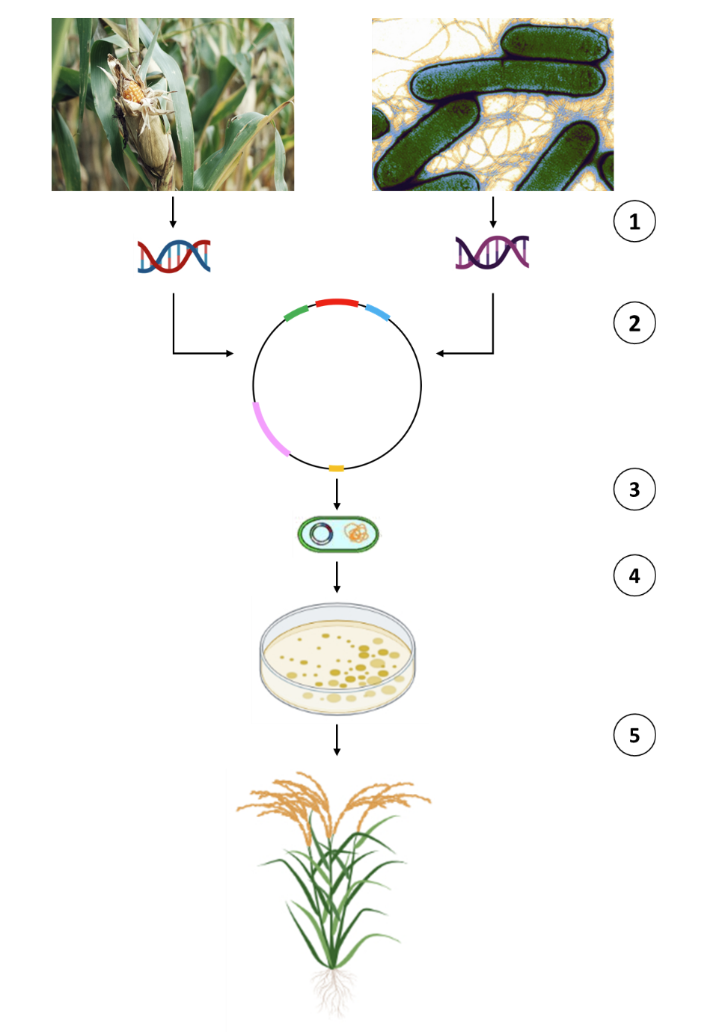
Figure 2: The production process of Golden Rice 2 (SGR2) developed by agricultural biotechnology firm Syngenta. The crucial steps involved: (1) Essential genes for β-carotene production were taken from maize and the P. ananatis bacterium, and (2) these genes were inserted into Ti plasmids. (3) Ti plasmid is then inserted into A. tumefaciens that introduces the modified plasmid into rice cells by (4) mixing them with rice embryos in Petri dishes. (5) The genetically modified rice embryos (now containing the carotene genes) were grown into mature adult rice plants, which can produce β-carotene-containing seeds in their endosperm. Figure created on BioRender.
Why should you care?
The lack of even distribution for food security across the world makes the Golden Rice invention an ingenious creation, as it is used to help populations where Vitamin A deficiency (VAD) is prevalent.
Golden Rice does not come as a single rice variety as farmers can cross them with the common rice varieties in their countries by conventional breeding so that the rice plants will possess the same traits as the local rice varieties but with an enhanced provitamin A content. Considering that rice is a staple food in most of the identified countries, the Golden Rice offers a diet with an increased vitamin A intake that can reduce the childhood mortality rate by 54%.
Estimations indicated that one million child deaths could be prevented annually if their Vitamin A intake were improved. Taking India for example, the adoption of Golden Rice is projected to have its most significant impact among poorer households, whereby it could reduce the Vitamin A intake gap in women by 25% and children by 11%. This would ultimately result in a 59% decrease in VAD's burden and save 40,000 lives every year. Another case study on the impact of Golden Rice in the Philippines suggested a 6 to 47% reduction in the proportion of DALYs (disability-adjusted life years) lost each year by implementing the Golden Rice project. Thus, this leads to a 0.09 to 0.53% increase in labour productivity among unskilled workers. Meanwhile, the national income of China is estimated to rise by 2% as the cultivation of Golden Rice is implicated in improving the national welfare gains and alleviating poverty significantly.
What's in line for us?
Biotechnology holds a viable solution that aligns with the United Nations' Millennium Development Goals in eradicating extreme hunger, reducing child mortality, and improving maternal health.
Although it is not a panacea for solving the global issue of food resources, genetic engineering techniques such as Agrobacterium-mediated transformation can indirectly help to resolve the global problems through its impact on public health, economic status and individual welfare in a sustainable way.
Author: Bianca Khor, BSc Biochemistry
Disclaimer: All figures created using BioRender are intended solely for educational purposes and not for profit.
Comments