Viral Content: An Introduction to Virology
- Ambar Khan
- Aug 17, 2020
- 10 min read
Updated: Jul 16, 2023
What is a virus?
You would have probably heard of the three domains of life - eukaryotes, archaea and bacteria. Viruses, however, are not members of any of these groups as they are not considered to be alive (although there is some debate regarding this).
Instead, viruses are defined as obligate, intracellular parasites that can infect any cells, originating from various types of organisms ranging from animals to plants to bacteria, and even yeast to fungus. Interestingly, some viruses, known as ‘virophages’, which infect other viruses have even been discovered recently.
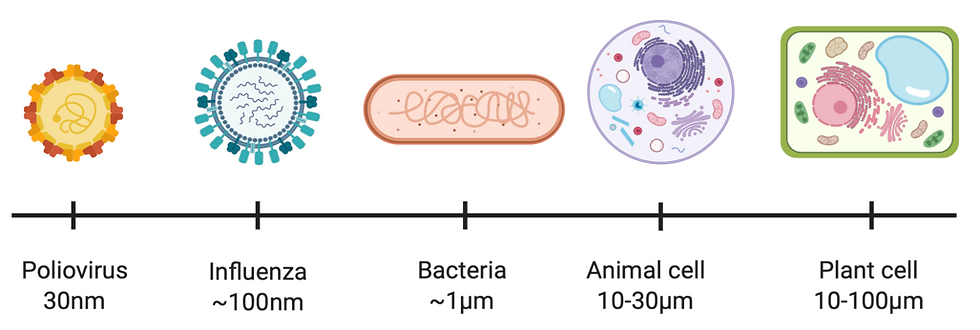
Figure 1: As shown by the scale, viral particles are much smaller than cells. In fact, they cannot even be seen under a light microscope. Figure created on BioRender.
The discovery of viruses
Louis Pasteur and Edward Jenner made a name for themselves by discovering some of the first vaccines against viruses (which were rabies and smallpox, respectively). Despite these huge breakthroughs, neither of them actually knew what a virus was.
It wasn’t until the later experiments whereby the nature of these infectious agents was actually determined. In 1892, Dmitry Ivanovsky passed an extract from plants with tobacco mosaic disease through a filter, in which bacteria couldn’t fit through. The resulting filtrate was still capable of causing tobacco mosaic disease in plants, so this causative pathogen was known for a long time as a ‘filterable infectious agent’.
The work of many scientists from the 19th century onwards allowed us to build on these first observations. In 1910, Nobel-winner Peyton Rous discovered the first oncogenic (meaning cancer-causing) virus, rous sarcoma virus; which causes sarcomas in chickens. Meanwhile, the tobacco mosaic virus became the first virus to be crystallised in 1935 by Wendell Stanley; this won him a Nobel Prize as this research was vital for our understanding of the basic structure of a virus. This is particularly important given that viruses are too small to be seen or studied under a light microscope.
The structure of a virus
A virus consists of a capsid (another name for a protein coat) which surrounds the nucleic acid that encodes their genome. However, there is a huge amount of variation around this basic structure.
Firstly, the capsid shape can be either icosahedral, helical or complex (as seen in Figure 2). Secondly, some viruses, such as the influenza virus, can be further surrounded by an envelope known as the outer layer. This layer is derived from the lipid bilayer membrane of the host, and different types of viruses would have different types of proteins embedded within their own unique outer layers.
Virion particles (meaning the complete form of the virus) can also vary in the number and type of proteins they contain. Poxvirus virions, for example, are unique in that they carry their own RNA polymerase, an enzyme that uses the DNA genome as a template to produce RNA transcripts (which are subsequently translated into proteins by host ribosomes). In contrast, the retrovirus HIV virion carries a reverse transcriptase protein - this converts the virus’s RNA genome into DNA so it can be integrated into the hosts genome.
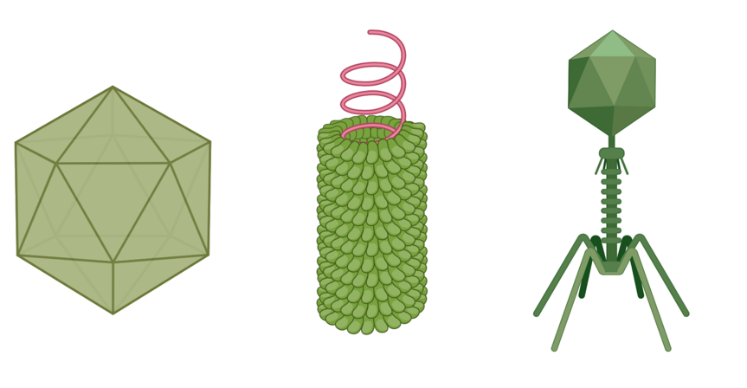
Figure 2: A virus capsid can either be icosahedral (left), helical (centre), or complex (right). Figure created on BioRender.
An overview of the viral genome
As well as structural variation, the nucleic acid making up a virus’ genome can differ between different viral species.
Whilst some viruses consist of double-stranded DNA (See Figure 3a) – something you would have probably come across frequently when studying living organisms – viral genetic material is most commonly made of single-stranded RNA (Figure 3d). Alternatively, viruses can have single-stranded DNA (Figure 3b) or double-stranded RNA genome (Figure 3c).

Figure 3: Viral genomes can be made up of (a) double-stranded DNA, (b) single-stranded DNA, (c) double-stranded RNA or (d) single-stranded RNA.
Meanwhile, it comes with no surprise to learn that viral genomes are (almost always) smaller than the genome of cellular organisms. However, just like their structure and nucleic acid content, the size of a viral genome can also vary greatly from virus to virus.
For instance, the smallest genomes ever recorded belongs to the virus Phi-X174, which is made of only 5386 nucleotides; whilst the Pandoravirus currently holds the record for the largest viral genome, at ~2.8Mbp!
The life cycle of a virus
Although the life cycle of a virus can differ as a result of a variety of factors – such as whether or not the virus has an outer envelope - there are five steps typically seen in their life cycle:
1. Attachment
The virus binds to a specific receptor on its host cell
2. Penetration/entry
The virus enters the cell or injects its genetic material
3. Genome replication and gene expression
The virus hijacks host cellular machinery, such as ribosomes and polymerases, forcing the cell to become a ‘virion factory’. As a result, viral proteins can now be produced, and its genome can be replicated
4. Assembly
Newly synthesised viral proteins and genome copies self-assemble to form new virion particles
5. Release
Virion particles leave the cell and can infect other cells
What do viruses infect?
We know that viruses can infect all living organisms, so let’s look in more detail at a few of these different hosts and the type of viruses they can get infected with:
Animals
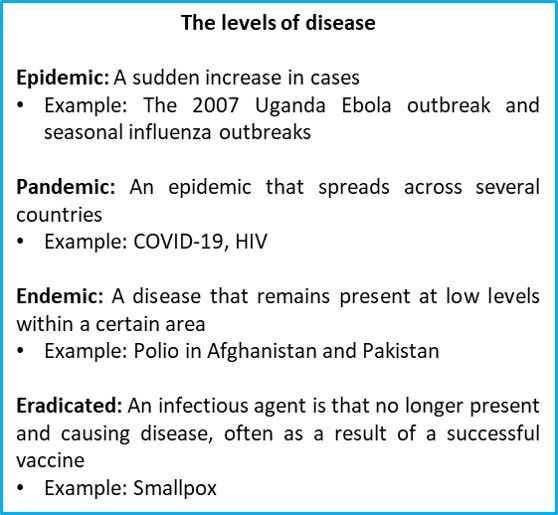
Figure 4: Both infectious and non-infectious diseases can be assigned an epidemiological level based on their prevalence in an area.
Some of the worst epidemics in the history of mankind have been caused by these seemingly simple infectious agents.
Smallpox is estimated to have killed as many as 500 million people in the 20th century alone. Besides that, the Spanish Flu of 1918, which was an outbreak of the influenza virus, lasted for only a year but was responsible for 50 million deaths. And unless you have been living under a rock, we all know from the global COVID-19 pandemic that viral outbreaks are not a thing of the past.
A number of diseases in humans are zoonotic, meaning that they are a result of a cross-species transmission event from animal to humans (have a look at Figure 3). This is because viruses have a relatively high mutation rate, allowing them to adapt to a new host species relatively easily.
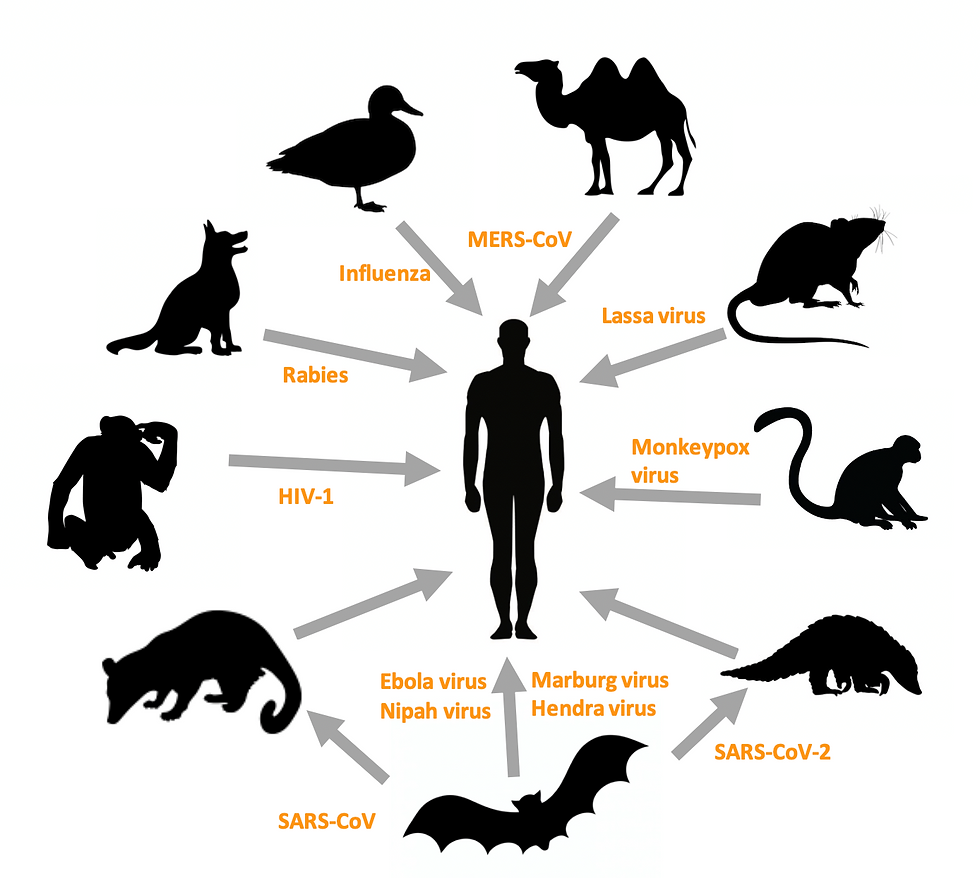
Figure 5: Cross-species transmission events of viruses from their main host reservoir to humans, resulting in zoonotic diseases. In some cases, the virus may have more than one main reservoir (not shown in this diagram), SARS-CoV and SARS-CoV-2 (COVID-19) were both transmitted to humans from bats via palm civets and armadillo, respectively. Important abbreviations in this diagram: MERS-CoV = Middle Eastern Respiratory Syndrome Coronavirus; SARS = Severe Acute Respiratory Syndrome. Figure created on BioRender.
Each animal virus will have at least one cognate receptor that is recognised by the host cell. This recognition determines a virus’ tissue tropism – meaning the cells and tissues in the host organism which the virus can infect and multiply in – and therefore the symptoms of the disease.
For example, HIV (human immunodeficiency virus) can infect any cells with the CD4+ receptor on their surface, under the condition that these cells also have either one of these two receptors too, the CCR5+ or CXCR4+ co-receptor. If the combination of these two receptors are present, this means HIV can enter and kill our white blood cells (namely the T helper cells or macrophages) and thus cause immunosuppression within their victims.
Another interesting characteristic of some animal viruses is their ability to remain dormant when infecting a host cell- this is known as the latent stage of infection. Latency occurs when a particular virus enters a cell and integrates its viral DNA into the host cell’s genome, thus becoming a provirus (a provirus is defined as an inactive form of a virus that has integrated its genetic material into the host’s DNA). It then replicates as the cell divides and is inherited by daughter cells, whilst remaining undetected by the host’s immune system.
Going back to our case study on HIV, HIV can infect CD4+ cells latently. This means that it can never be completely cleared from the patient’s body, so this makes HIV infections incurable for the time being. The only treatment available is ART (anti-retroviral therapy), which are basically drugs that keep the viral load low enough in order to prevent the disease from progressing to AIDS (acquired immunodeficiency syndrome). Similarly, herpes simplex virus-1 infects and causes cold sores before becoming latent. Therefore, the reactivation of this virus is responsible for reoccurrence of cold sores. On the other hand, the wide-spread cytomegalovirus, another herpes virus, is present in almost 50% of adults and remains latent in the bone marrow. Even though those who are infected are often asymptomatic, the pathogen can still be transmitted in utero and causes congenital defects in 1% of live births.
Plants
Plants are also susceptible to infection by a huge range of viruses.
The presence of a cell wall in plant cells means there are a number of differences in the lifecycle and transmission of these viruses when compared to viruses that infect animals.
For example, plant viruses cannot simply bind to a cell to infect it. Instead, they must wait for the plant to become damaged so that the cell wall can be breached, or alternatively, rely on a vector to transmit it. A vector is an organism which does not cause disease, but instead spreads a pathogen from host to host. Interestingly, some viruses can be transmitted via seeds to the offspring of an infected plant.
Plant viruses are unique in that they are not specific to a single species. This means that a single virus can infect a range of related plants. For instance, members of the family Solanacaea, including tobacco, tomatoes and potatoes, are all susceptible to infection by the well-studied tobacco mosaic virus. Meanwhile, the Cauliflower mosaic virus is vectored by aphids and infects broccoli, cauliflower, turnips and other members of the Brassicaceae family.
Bacteria
Viruses that infect bacteria are called bacteriophages. Bacteriophages can be classified as virulent or temperate depending on whether they undergo a lytic or lysogenic cycle, respectively. In essence, the lytic cycle results in death of the host cell and release of new viral progeny. On the other hand, in the lysogenic cycle, the host cell is not killed, but the virus is incorporated into its genome.
Take a look at Figure 6 to help you picture these two scenarios.
Virulent bacteriophages undergo a lytic cycle
Virus attaches to a host bacterial cell
Virus penetrates the cell and injects its genetic material into the host
The viral genome is transcribed, translated and replicated by host machinery
New bacteriophages assemble and accumulate
Eventually, the host cell lyses (bursts) and the new bacteriophages set out to infect other cells
Temperate bacteriophages undergo a lysogenic cycle
Virus attaches and penetrates cell just like how it would during a lytic cycle
Virus integrates its genome into the host DNA and become a prophage (meaning an inactive form of the bacteriophage). This step is process is similar to that of some animal viruses when they latently infect and become proviruses
As the host cell replicates its DNA and divides, the phage will be passed on to the daughter cell
Prophage induction takes place, meaning that the phage DNA can be excised and enter the lytic cycle as a result of that
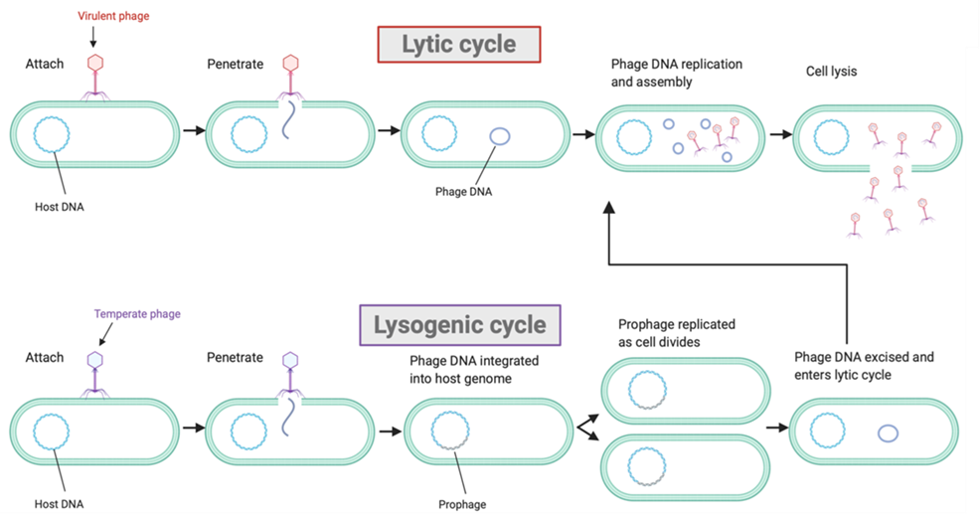
Figure 6: Bacteriophages can undergo either the (1) lytic or (2) lysogenic cycles when infecting their bacterial host cell. Figure created on BioRender.
Why is it so important to study viruses?
Now you know some of the basics of virology, it’s good that we also have a think about the importance of viruses and why we need to study them.
The cost to human life
As we’ve already discussed, viruses are responsible for various human diseases, many of which have caused and are yet to cause a significant loss of lives.
Aside from epidemics, viruses are also responsible for a proportion of cancers. HPV (human papillomavirus), for example, causes 91% of cervical cancer cases. Measles, rabies, polio, hepatitis, influenza, chickenpox, common colds and many more are all caused by viruses that are still present in the modern era. As a matter of fact, in 2019, it was estimated that 38 million people are still living with the incurable HIV.
It is therefore vital that we learn as much as we can about these infectious agents so we can continue to prevent epidemics, develop treatments and vaccines and eradicate these diseases.
The economic cost
Plant viruses can cause devastating losses to crop yields. Due to their reliance of agriculture for income and food security, developing countries are often disproportionately affected. For example, in the sub-Saharan areas of Africa where banana bunchy top virus is present, many farmers face a 70-90% reduction in their crop yields thanks to viruses. More specifically, once plants are infected, they cannot be treated.
By understanding the transmission of plant virus’ and their mechanism of disease, we can reduce the economic cost these diseases have by finding methods to treat plants and prevent infection in the first place.
The benefits
Although viruses are infectious agents, it is possible to take advantage of their unique lifestyle for our own benefits.
One example of this is in viral gene therapy. This is used to treat genetic diseases, which are caused by defects in an individual’s genome, such as cystic fibrosis and sickle cell anaemia. The normal form of the faulty gene is inserted into a viral vector, which has been modified to not cause diseases, and then delivered to the patients so that they can produce the functional protein.
Secondly, oncolytic viruses preferentially infect and kill tumour cells. Therefore, they have the potential to be used as a form of immunotherapy to treat cancer.
Bacteriophages have been proven to be extremely useful to molecular biologists. In particular, the temperate lambda phage has been used as a vector to transfer DNA between bacteria. Other than that, some bacteriophages are used in ‘phage display technology’, which involves inserting foreign DNA into the phage genome and so can be used to produce specific monoclonal antibodies. Monoclonal antibodies are antibodies that are all produced by B-cell clones (meaning that the B cells have all descended from one parent cell) and recognise the same binding site. These could have therapeutic benefit, for example, they could be used in vaccines against HIV or prostate cancer.
Another very successful use of viruses is as biological controls of fungal infections. Multiple chestnut blight epidemics had almost driven the American Chestnut to extinction, until a hypovirus, which infects the causative fungus and reduces its virulence, was discovered. Introduction of the virus into affected regions helped to limit the spread of chestnut blight, and without it the fate of the American Chestnut could have been very different.
Author: Ambar Khan, BSc Biological Sciences
Disclaimer: All figures created using BioRender are intended solely for educational purposes and not for profit.